How to unwire optical implants and even displays
Researchers are using a new method to switch a light source required for optogenetic analyses even in centimeter-deep tissue layers without cables.
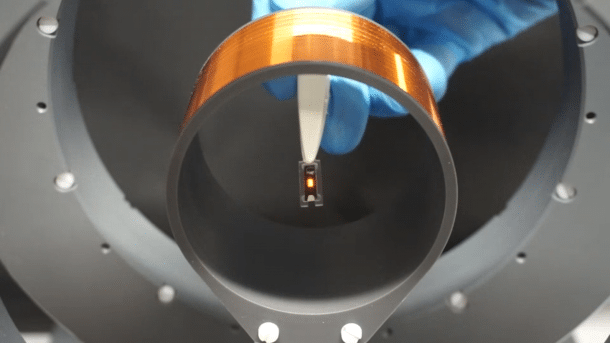
The ME-OLED, which is held by plastic tweezers, lights up as soon as it is exposed to an alternating magnetic field. To achieve this, the team combines magnetostriction with piezoelectricity in a sandwich-like structure.
(Image: Julian Butscher et al)
Unlike the ocular implants used by Borgs and other sci-fi creatures to give them a menacing appearance, optical implants are used in real life to analyze cells and tissues, among other things: In genetically engineered cells, certain functions can be switched with light pulses depending on time and location. Among other things, groundbreaking studies on neuron activity are based on this. However, the light sources developed to date based on inorganic light-emitting diodes (LEDs) are bulky and draw the required current via cables, which impairs model organisms and prevents some experiments.
In principle, the required energy can be transmitted wirelessly in various ways. However, even the wireless methods developed to date have disadvantages: For example, an ultrasound transducer requires direct contact with the tissue to avoid reflections, and the ultrasound signal can heat the tissue considerably. Devices based on other methods are too large or only cover a few millimeters.
A research team from the University of St Andrews in Scotland and the University of Cologne has now achieved breakthroughs in both miniaturization and range: A conventional coil pumps energy into space in the form of an alternating magnetic field, which is absorbed by a thin-film antenna made of the magnetostrictive material Metglas. Metglas is a metallic, non-crystalline, rubber-like alloy that stretches and compresses according to the changing direction of the magnetic field. The Metglas transmits its movements to a piezoelectric layer of lead zirconate titanate bonded with epoxy resin. And this second layer converts the mechanical movements into electrical voltage – and the wireless power supply is complete.
Antiparallel sandwich
The highlight, however, is that this magneto electric double layer (ME) is suitable as a carrier for organic light-emitting diodes (OLEDs). The team led by Professor Malte Gather uses this for a sandwich-like structure: they apply organic light-emitting material to both sides of the magneto electric double layer and thus form a magneto electric organic double LED (ME-OLED). As the diodes are connected antiparallel to the power source, they utilize both halves of the magnetic sinusoidal signal. The bottom line is that the power receivers, converters and cables required for inorganic LEDs are completely eliminated.
The team chose low-frequency alternating magnetic fields around 100 kHz for the power supply. This allows an ME-OLED to be operated several centimetres deep in an aqueous environment. The energy is transmitted via the resonant frequency of the ME antenna, which results in further advantages. As with other antennas, the resonant frequency depends directly on the length of the ME antenna. Therefore, ME-OLEDs can be switched separately even in close proximity if there is a sufficient difference in length - thus fulfilling the requirement for a display consisting of several pixels.
The ME-OLED signal has a width of around 7 kHz, so that 28 elements can be addressed in a 100 kHz wide band at a distance of 3.5 kHz. The group initially implemented the concept with three ME-OLEDs and resonant frequencies of 130.5, 139 and 149 kHz.
As with conventional antennas, the higher the frequency band, the shorter the dimensions of the ME antennas. For the range from 100 to 200 kHz, lengths of 14.9 to 7.4 millimeters are required. In the 400 to 500 kHz band, 3.7 to 3 millimeters are required.
The researchers are convinced that their method will pave the way for a wide range of applications in the fields of wireless displays, deep tissue treatment, sensor technology, imaging and medicine. This is probably one of the reasons why first author Julian Butscher and Professor Gather applied for a patent for the technology on behalf of the University of Cologne at an early stage, which the university has now held since August 2023. The study was published in the journal Science Advances under the title "Wireless Magnetoelectrically-Powered Organic Light-Emitting Diodes".
(dz)